Electron
The electron
The following text is used only for teaching, research, scholarship, educational use and informative purpose following the fair use principles.
We thank the authors of the texts and the source web site that give us the opportunity to share their knowledge
Physics
The electron
'The electron is a theory we use; it is so useful in understanding the way nature works that we can almost call it real.'
Nobel Prize winning physicist Richard Feynman
Properties of the electron
- Orbits the nucleus
- Very small mass
- Negatively charged
- The charge on the electron is the smallest amount of charge found in nature*.
You don’t have to know the actual charge (1.6 × 10-19 C), but you do need to know that the man responsible for first measuring this charge was Robert Millikan.
The term electron was coined by an Irishman called George Stoney.
Thermionic Emission is the giving off of electrons from the surface of a hot metal.
The Cathode Ray Tube*
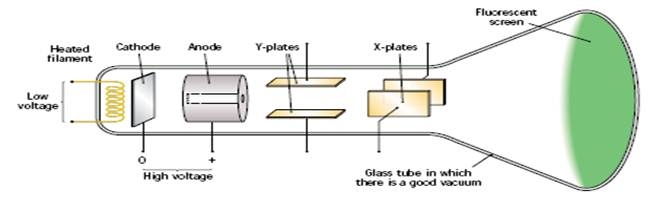
Operation
- A current is passed through the heating coil, causing it to heat the cathode which in turn causes electrons to be emitted (by thermionic emission).
- Because of the high potential difference between the cathode and anode the electrons are accelerated across the tube towards the anode. As a result a current flows in the circuit.
- Electrons which pass through the hole in the middle of the anode continue on until they hit the fluorescent screen.
- The stream of electrons can be deflected by electric or magnetic fields, which are generated from the X and Y plates.
- The voltage on the Y-plates is adjusted to make the cathode rays (or stream of electrons) move up or down.
- The voltage on the X-plates is adjusted to make the cathode rays move left or right. The result is that the rays can move across the computer or television screen.
Uses of the Cathode Ray Tube
Basically it can be used anywhere a small electrical signal is produced.
- Cathode Ray Oscilloscope (CRO).This is a variation of the cathode tube; it is used today in electronics as a diagnostic tool.
- It also forms the main component in televisions and computers*.
- The ECG (electrocardiogram) is used in medicine to display electrical signals in the heart.
Alternatively it can be used to display signals produced by the brain, where it is known as an EEG (electroencephalogram).
Cathode rays are streams of high-speed electrons
Properties of cathode rays
- They travel from the cathode in straight lines
- They cause certain substances to fluoresce
- They can be deflected in electric and magnetic fields
- They can produce x-rays when they strike heavy metals
Energy associated with an electron*
An electron’s potential energy (W= eV) can be converted to kinetic energy (W = ½ mv2)
W = QV
W = eV
eV = ½ mv2
This leads us to the concept of the electron-volt:
The Electron Volt (eV) *
The Electron Volt (eV) is the energy lost or gained by an electron when it accelerates through a potential difference of one volt.
From the formula W = eV, we can calculate that the energy lost or gained is (1.6 × 10–19)(1), or 1.6 x 10–19 Joules
1eV =1.6 x 10–19 Joules
See Worked Problems 3 and 4, page 331. Then try questions 4 and 5, page 333.
Electrons in a magnetic field
A beam of electrons moving at right angles to a magnetic field will move in a circular path*.
Look over the Worked Problems on page 330. Then try Questions 1, 2, 3, page 333.
You should also revise questions from page 236 at this stage.
We know (don’t we?) that the force on a charge q moving at velocity v in a magnetic field B is given by
F = Bev
F = mv2/r
mv2/r = Bev
Now putting these two together we get
Where m is the mass and r is the radius of the circle.
By rearranging this expression we can find the radius of orbit if we know everything else.
Note for each of the previous three equations v = velocity, not voltage
Now look at worked solutions 5, 6, 7 page 332 and then try Questions 6 – 11, page 333.
The Photoelectric Effect
The Photoelectric Effect is the emission of electrons from a metal due to light of a suitable frequency falling upon it.
Demonstration
Procedure:
- Charge the electroscope negatively.
- Shine ultraviolet light on the zinc plate.
Result:
The leaves fall together
Observation:
Shining UV light on the zinc plate liberates electrons from the zinc and therefore the legs become neutralised and fall back together.
Einstein’s Explanation
- The incident energy is absorbed by an electron at the surface of a metal.
- A certain amount (the work function) goes to liberating the electron.
- The remainder appears as kinetic energy of the liberated electron*.
- Shining visible light has no effect on the apparatus.
This is because the packets of energy associated with visible light are too small to liberate electrons.
This “proved” that electromagnetic radiation (including light) is composed of particles (called photons).
A photon is a bundle (discrete amount) of electromagnetic radiation.
Mathematically: the photon as a packet of energy*
E = hf
The energy associated with each packet of energy (photon) is given by
h is known as planck’s constant and f is the frequency of the wave.
According to Einstein's equation for the photoelectric effect (also known as Einstein’s photoelectric law):
Energy of incident photon = work function + kinetic energy of photo-electron.
hf = f + ½mv2
f (“phi”) is known as the work function.
It represents the energy required to ‘liberate’ an electron from the surface of a metal.
Because f is a discrete amount of energy, it can in turn be represented by the formula f = hf0, where f0 is called the threshold frequency.
The value of the work function is different for different metals.
The Photocell
Operation
- Light of a suitable frequency shines on A (called the photocathode).
- This releases electrons (by the Photoelectric Effect).
- The electrons are attracted to point B (the anode), and from there they flow around the circuit, where they can be detected by a galvanometer, or alternatively they can be used to activate an electronic device.
Applications of photoelectric sensing devices
- Burglar alarms
- Automatic doors
- Control of burners in central heating
- Sound track in films
X-Rays
X-rays are produced when high-energy electrons collide with a high density target.
Operation of the X-ray tube
- The low voltage supplies power to a filament which in turn heats the cathode at A.
- Electrons are emitted from the hot cathode due to Thermionic Emission.
- They get accelerated across the vacuum due to the very high voltage and smash into the high-density anode (usually tungsten) at B.
- Most of the kinetic energy gets converted to heat, which must be removed with a coolant.
- Some inner electrons in the tungsten get bumped up to a high orbital, then quickly fall back down to a lower lever, emitting X-rays in the process.
- These X-rays are emitted in all directions.
- Most of these get absorbed by the lead shielding, but some exit through a narrow window, where they are then used for the required purpose.
Properties of X-Rays:
- They are Electromagnetic Waves
- They cause ionisation of atoms
- They have high penetration powers
Uses of X-rays
- Medicine: To detect broken bones
- Industry: To detect breaks in industrial pipes
Why can X-ray production be considered as the inverse of the Photoelectric Effect?
Hazards*
They can ionise atoms in the body, causing them to become abnormal, which can lead to cancer.
Leaving Cert Physics Syllabus
Content |
Depth of Treatment |
Activities |
STS |
|
|
|
|
1. The electron |
The electron as the indivisible quantity of charge. |
|
Electron named by G. J. Stoney. |
|
|
|
|
2. Thermionic |
Principle of thermionic emission and its application to the production of a beam of electrons. Cathode ray tube consisting of heated filament, cathode, anode, and screen. Deflection of cathode rays in electric and magnetic fields. |
Use of cathode ray tube to demonstrate the production of a beam of electrons – deflection in electric and magnetic fields. |
Applications |
|
|
|
|
3. Photoelectric |
Photoelectric effect. |
Demonstration, e.g. using zinc plate, electroscope, and different light sources.
Demonstration of a photocell. |
Applications of photoelectric |
|
|
|
|
4. X-rays |
X-rays produced when high-energy electrons collide with target. |
|
Uses of X-rays in medicine and industry. |
Extra Credit
About one hundred years ago, several remarkable and highly-important discoveries were crowded into the short space of ten years: X-rays in 1895, radioactivity the following year, the electron in 1897, quantum theory in 1900, and special relativity in 1905.
Individually, each had enormous significance and collectively they heralded what is now known as ‘modern physics’.
*The charge on the electron is the smallest amount of charge found in nature
Physicists still don’t know why an electron has the same charge as a proton!
Later on we will come across particles called quarks, some of which have a charge of 1/3rd that of the electron. But these are not found isolated in nature.
*The Cathode Ray Tube
This phenomenon was noticed before people were familiar with the concept of the electron.
What was known was that if a fluorescent screen was placed on the inside of the tube, fluorescence would occur.
Therefore something seemed to be coming from the cathode.
Because the electrons could not be seen, this ‘something’ was called cathode rays, and the apparatus was called a cathode ray tube.
Incidentally, the cathode ray tube was first developed by Sir William Crookes, of Crooke’s Radiometer fame (remember what that is?). Crooke was a major player in the world of physics in the late 19th century.
Crooke later lost all respectability among his peers due to his willingness to get involved in physic research (being able to read someone else’s mind and all that). Crookes' final report so outraged the scientific establishment that there was talk of depriving him of his Fellowship of the Royal Society.
*The Cathode Ray Tube forms the main component in televisions and computers.
For this the screen is divided up into little squares called ‘pixels’ (from ‘picture elements’).
The ‘electron-gun’ scans across each row of pixels, starting at the top left hand corner and working down, changing intensity as it travels.
Each pixel stays bright for a fraction of a second, but before it starts to fade the gun has gone back over it and ‘refreshed’ it.
The quality of a television screen is therefore determined by, among other things, the speed at which the gun travels, and the number of pixels on the screen. For example, a 640-by-480 pixel screen is capable of displaying 640 distinct dots on each of 480 lines, or about 300,000 pixels.
For colour televisions there are actually three ‘guns’ involved, each having one of the three primary colours, and the product of their relative intensities determine the colour seen on a given part of the screen.
‘Course by the time you read this the cathode ray tube will probably be obsolete.
*Energy associated with an electron
When an electron gets accelerated across a potential difference, it gains kinetic energy (½ mv2).
But just like an apple falling from a tree which similarly gains kinetic energy, this energy had to come from somewhere.
In the case of the falling apple, the energy it gained came at the expense of (Gravitational) Potential Energy (mgh) which it lost.
In the case of the electron the kinetic energy gained comes at the expense of Electrical Potential Energy, the formula for which is W = QV. Therefore we end up with the expression eV = ½ mv2.
If any of this seems vaguely familiar it’s because we studied it in chapter 20.
In this case Q represents the charge on an electron, for which we use the letter e. So we get W = eV.
Now the problems on page 330 are similar to those on page 236, but should still be studied before trying Questions 1, 2, 3, page 333.
*The Electron Volt (eV)
When dealing with energies in our everyday world, we use the joule as the unit of energy (can you remember the definition of the joule? Hint: think of the formula for work).
When working in the world of electrons however, the energies involved are so small that rather than using the joule, another unit is more commonly used. This unit is called the Electron Volt.
*A beam of electrons moving at right angles to a magnetic field will move in a circular path.
A beam of electrons constitutes an electric current but remember that if a current flows in one direction the electrons are actually moving in the opposite direction (and vice versa).
Now if an electron is moving towards the right (meaning current is to the left) in a magnetic field where the direction of flux density is into the page, applying Fleming’s Left Hand Rule tells us that the force on the electron must be downward, and so the electron changes direction.
But because the flux density is constant and is always perpendicular to the direction of the electron, the electron will continually change direction, while moving at the same speed.
The result is that it travels in a circular path.
We’ve come across this concept before, i.e. something which is travelling at constant speed, but changing direction is accelerating.
Why is the flux density always perpendicular to the direction of the electron?
Because the flux density is always into the page, while the electron is always travelling along the page, even though it’s changing direction on the page.
This is one of those concepts that I would be afraid of tackling if it came up on an exam question. You never know how much detail is required, and the chances are that the marks are only awarded for two or three key phrases, all of which may or may not have been included in the notes above.
*The photon as a packet of energy;
In 1905 Einstein published a famous paper that suggested that light could be considered to consist of packets, which he called photons.
Max Planck was the dude who kick-started all this Quantum Theory stuff ten years previously by suggesting that heat energy could be quantised.
Funnily enough, he could never accept Einstein’s findings (which followed on from Planck’s work) that all energy could be considered to consist of discrete packets.
But this was only the beginning of Planck’s misfortune.
To get an idea of how poor a hand he got dealt in life, read Bryson’s account of it in his book A Short History of Nearly Everything.
One of the consequences of Einstein’s work was that it was realised that there was a randomness inherent in the laws of physics at the quantum level. Ironically Einstein could never accept this.
His famous phrase was “God does not play dice”.
For what it’s worth, it is a common misconception that work-function is the same as ionisation energy.
Ionisation energy involves bumping electrons out of single standalone atoms (or perhaps molecules) in a gas.
In a metal there is a ‘sea of electrons’ on the surface, and it is one of these electrons which leaves the surface when light of a suitable frequency falls on it.
Because the electron arrangement is different in both cases, the energy involved will be different.
Coining of the term ‘Photon’.
"I therefore take the liberty of proposing for this hypothetical new atom, which is not light but plays an essential part in every process of radiation, the name photon."
Gilbert N. Lewis, 1926
Incidentally Einstein's description of the quanta of energy of EM radiation first used 100 years ago still serves as an excellent definition of a photon to this day:
“Energy during the propagation of a ray of light is not continuously distributed over steadily increasing spaces, but it consists of a finite number of energy quanta localised at points in space, moving without dividing and capable of being absorbed or generated only as entities.”
*The Photoelectric Effect
The incident energy is absorbed by an electron at the surface of a metal.
A certain amount (the work function) goes to liberating the electron.
The remainder appears as kinetic energy of the liberated electron.
The strange thing is that if the incident light hasn’t got enough energy to liberate an electron, no amount of time (or increase in intensity) will make a difference.
An increase in intensity simply means that more packets of light are used per second, but if they don’t have sufficient energy, increasing the number will simply have no effect.
However if each photon has enough energy to liberalise an atom, increasing the intensity (i.e. the number of photons) will result in more atoms being liberalised, and therefore an increase in current.
Remember that the energy of the incident light is determined by its frequency.
So for example for the metal zinc, visible light is of too low a frequency, and therefore has too little energy, to liberalise electrons form the surface of the zinc.
However Ultra Violet light has a higher frequency and therefore is able to liberalise electrons.
This can be detected as a small current using an ammeter or galvanometer.
This proves that light is like a particle.
It was Einstein who realised this.
Analogy of the Photoelectric Effect
The energy of the incident photon is like the money in your pocket at the start of a night out
The work function energy is analogous to the admission charge at the nightclub.
It's an all or nothing thing, even if you're only just one penny short, you will be refused entrance.
Different clubs may charge different entrance fees, which is analogous to the differing work functions of different metals.
The maximum Kinetic Energy bit represents the money left over after paying for entrance, which can then be spent on lemonade J
A nice analogy for work function, but as with all analogies it's worth discussing the limitations.
For instance if one of the group does not have quite enough money, but the rest have some to spare, they can all get in.
Photons, on the other hand, cannot re-distribute energy between themselves.
Even if a photon with insufficient energy arrives at the same time as others with excess energy, it still cannot eject an electron.
*X-Rays
X-Rays will prove to be a hoax
Lord Kelvin
Remember Kelvin’s remark on alternating current also being a mistake in the last chapter?
*Hazards
X-rays Radiation can cause the ionization of atoms in a persons’ DNA.
This means the atom is now charged, where before it was neutral.
This can affect the function of the DNA.
The DNA can be damaged and then repaired in such a way that the cell continues to divide but with an altered message in the DNA.
This altered message may eventually result in a cell turning into a cancer.
Thus any dose of radiation increases the risk of cancer.
Believe it or not, X-Rays used to be used to help in ascertaining the size and shape of a person’s foot; The customer put his or her foot in the machine for up to 45 seconds, while the salesperson had to use this machine all day.
Quantum Theory
“Curiouser and curiouser,” cried Alice
Alice’s Adventures in Wonderland
“The more successful quantum physics gets, the sillier it looks”
Albert Einstein
“No-one understands quantum physics”
Richard Feynman
Introduction to Quantum Theory
Planck started the whole thing off, saying that heat was given off as little packets as opposed to continuous waves.
Einstein took this a step further and suggested that perhaps light was also given off in little packets (called photons) instead of as a continuous wave.
Planck, however, thought this was preposterous and could never accept Einstein’s idea.
But Einstein also suffered a similar fate.
Predictability is one of the corner-stones of physics.
Resolving controversies in science usually involves successfully predicting the outcome of a specially-designed experiment.
But it turns out that in nuclear physics an atomic nucleus breaks down into other, smaller nuclei, and predicting when this will occur is impossible.
The best we can do is to give the probability of a break-down occurring.
Einstein, whose own work formed the foundation for these ideas, could never accept these conclusions.
In fact one of his most famous quotes was on this subject: “God does not play dice”. What he meant by this is that the universe was not built around laws of probability (the reference to God was not meant to be taken literally – Einstein did not believe in a personal God).
For the record, all scientists now accept that probability does indeed lie at the heart of physics.
It gets better.
When formulating the rules for General Relativity – probably his greatest work of all – Einstein had assumed that the universe was static. i.e. it was not expanding.
This was in keeping with the belief at the time.
But one of the outcomes of General Relativity was that the universe was expanding. Believing that he had made a mistake somehow, he introduced a ‘fudge factor’ into his equations which stabilised the universe.
When the deliberate error was later discovered (by a postgraduate student) Einstein described it as ‘the greatest mistake of my life’.
By this stage the inflationary universe was becoming accepted as the correct description of the universe, which in turn implied that the ‘Big Bang’ did indeed occur.
One of the many ironies in all this is that it is now thought that the universe may not go on expanding forever, but may indeed either stabilise or begin to contract again, resulting in the universe finishing in a ‘Big Crunch’.
Either way, Einstein’s fudge factor may not have been such a mistake after all.
Of course this was just when Einstein acknowledged he was wrong.
He also made a bit of a serious boo-boo when it came to trying to unify the four forces of Nature.
As a result of this he lost almost all respect among his peers, and spent the last decades of his career on a lone crusade which yielded nothing but the patronising sympathy of his fellow physicists.
Interestingly, Einstein seems to have paid very little attention to developments in physics (including the discovery of all the new particles) during these latter years
We will come across the concept of the four forces in more detail in the chapter on ‘Particle Physics’.
By the way, one of the biggest advocates of the ‘steady universe’ scenario was a scientist called Fred Hoyle. Hoyle died recently, but in yet another irony, actually coined the term ‘Big Bang’ when he used it in a derogatory fashion.
The nerds among you may claim that this isn’t actually an example of irony, but you know what I mean.
Proving the electron is a wave using the electron diffraction apparatus
Knowing the mass of an electron, De Broglie’s formula gave the wavelength, which could be verified using carbon as a diffraction grating.
Conversely, if you knew the wavelength of light going in, and measured the separation of the rings formed on the screen, you could calculate the spacing of the atoms in the material which was acting as the diffraction grating.
Bragg (Junior and Senior) won Nobel prizes for their work on using X-rays to determine the determine the internal structure of various crystals.
This is still a very important tool in chemistry today.
Crick and Watson were able to use the results of X-ray crystallography to determine the structure of DNA molecules, one of the most important achievements in biology of the last 100 years
The fact that they had to cheat to get a sneak look at these pictures is a story for another day, but while these boyos were collecting their Nobel prizes for their work, nobody was bothering to mentioning the name of the woman who had carried out the initial work; for the record her name was Rosalynd Franklin, and Watson eventually owned up to what happened in a famous book which he later wrote, called The Double Helix.
This book is available in the Physics Lab.
It’s famous because it was one of the first books which gave an accurate picture of how science operates, warts and all.
It portrayed the pettiness, jealousies and pressure which scientists experienced and indeed which they still experience today, as do workers in any other institution (even our school staff-rooms!).
The difference was that science was usually portrayed as being above all that pettiness; the scientists themselves were supposed to only be interested in furthering scientific knowledge.
The Thompson family
JJ Thompson is said to have ‘discovered’ electrons. He suggested that the streams of cathode rays were ‘negatively charged corpuscles’, and that these were smaller than the atoms of Hydrogen.
He won a Nobel Prize for his work in 1906.
In 1927 JJ’s son G.P. Thompson investigated the wave-like nature of electrons and was able to demonstrate that they diffract, and therefore must be considered as waves. He won a Nobel Prize for his work in 1937.
So the father won a Nobel Prize for proving that the electron was a particle, while his son won the prize for proving that it was a wave!
How do identify the chemistry teacher from the biology teacher from the physics teacher?
Ans: Ask them to draw electrons around a nucleus.
Chemistry teacher: Now an electron has a negative charge, so we're going to represent them as little pluses.
Biology teacher: Let's put in big smiley faces and colour them yellow . . .
Physics teacher: Draw an electron?!? Draw an electron?!? Have you not been listening to a word I said? What gives you the right to decide what an electron looks like!?
Boom Boom!
Which reminds me of the story of the professor who began the new term with the following words:
Right, today we're going to continue on with our lecture on the electron. Now who can remind us what an electron is?
Hand goes up.
It's am ... It's am, eh, am, oh I knew this before the break but I must have forgotten it.
Professor: That's just great, there are only two people in this world who can tell us what an electron is; we can't get in touch with the creator, and you've just gone and forgotten it.
Boom Boom
Exam questions
Planck constant = 6.6 × 10-34 Js; Speed of light = 3.0 × 108 m s-1;
Charge on electron = 1.6 × 10-19 C; Mass of an electron = 9.1× 10-31 Kg
- [2003][2003 OL][2005 OL][2006 OL][2008 OL] List two properties of the electron.
- [2005 OL] Name another subatomic particle.
- [2003] Name the Irishman who gave the electron its name in the nineteenth century.
- [2002 OL][2009 OL] What is thermionic emission?
- [2008] [2002 OL][2005 OL] Give two ways of deflecting a beam of electrons.
The Cathode Ray Tube
- [2009 OL] What are cathode rays?
[2005 OL]
- The diagram shows a simple cathode ray tube.
Name the parts labelled A, B and C.
- Electrons are emitted from A, accelerated across the tube and strike the screen. Explain how the electrons are emitted from A.
- [2002 OL]
- The diagram shows a simple cathode ray tube.
Name the parts labeled A, B, C and D in the diagram.
- Give the function of any two of these parts.
- [2009 OL]
Why is there a high voltage between A and B?
- [2002 OL][2005 OL][2009 OL]
Give a use of a cathode ray tube.
- [2007] How are electrons accelerated in a cathode ray tube?
- [2005 OL][2009 OL] What happens when the electrons hit the screen?
- [2003] What happens to the energy of the electron when it hits the screen of the CRT?
- [2003]
- An electron is emitted from the cathode and accelerated through a potential difference of 4kV in a cathode ray tube (CRT) as shown in the diagram.
How much energy does the electron gain?
- What is the speed of the electron at the anode?
After leaving the anode, the electron travels at a constant speed and enters a magnetic field at right angles, where it is deflected. The flux density of the magnetic field is 5 × 10–2 T.
Calculate the force acting on the electron.
- Calculate the radius of the circular path followed by the electron, in the magnetic field.
F = mv2/r Þ 3.0 ×10−13 = (9.1 × 10–31)( 3.75 × 107)2/r
Þ r = 4.3×10−3 m
The photoelectric effect
- [2010] What is thermionic emission?
- [2004 OL] Which one of the following is emitted from a metal surface when suitable light shines on the metal?
protons neutrons electrons atoms
- [2004] Distinguish between photoelectric emission and thermionic emission.
- [2008][2005][2003][2005 OL][2008 OL][2010 OL]What is the photoelectric effect?
- [2005][2006 OL][2009 OL][2010 OL]Give two applications of the photoelectric effect.
- [2003 OL][2009]What is a photon?
- [2008]Why as the quantum theory of light revolutionary?
- [2002][2010 OL]Describe an experiment to demonstrate the photoelectric effect.
- [2005][2002]Outline Einstein’s explanation of the photoelectric effect.
- [2005]Write down an expression for Einstein’s photoelectric law.
- [2008]High-energy radiation of frequency 3.3 × 1014 Hz is used in medicine.
What is the energy of a photon of this radiation?
- [2004]
- Zinc metal is illuminated with ultraviolet light of wavelength 240 nm. The work function of zinc is 4.3 eV. Calculate the threshold frequency of zinc.
- Calulate the maximum kinetic energy of an emitted electron.
- [2004]
- A freshly cleaned piece of zinc metal is placed on the cap of a negatively charged gold leaf electroscope and illuminated with ultraviolet radiation.
Explain why the leaves of the electroscope collapse.
- Explain why the leaves do not collapse when the zinc is covered by a piece of ordinary glass.
- Explain why the leaves do not collapse when the zinc is illuminated with green light.
- Explain why the leaves do not collapse when the electroscope is charged positively.
- [2006 OL]
In an experiment to demonstrate the photoelectric effect, a piece of zinc is placed on a gold leaf electroscope, as shown. The zinc is given a negative charge causing the gold leaf to deflect.
- Explain why the gold leaf deflects when the zinc is given a negative charge.
- Ultraviolet radiation is then shone on the charged zinc and the gold leaf falls. Explain why.
- What is observed when the experiment is repeated using infrared radiation?
The photocell
[2003 OL]- The diagram shows a photocell connected in series with a sensitive galvanometer and a battery.
Name the parts labelled A and B.
- What happens at A when light falls on it?
- What happens in the circuit when the light falling on A gets brighter?
- [2008 OL]
A photocell is connected to a sensitive galvanometer as shown in the diagram.
When light from the torch falls on the photocell, a current is detected by the galvanometer.
- Name the parts of the photocell labelled A and B.
- How can you vary the brightness of the light falling on the photocell?
- How does the brightness of the light affect the current?
- [2009]
An investigation was carried out to establish the relationship between the current flowing in a photocell and the frequency of the light incident on it. The graph illustrates the relationship.
- Draw a labelled diagram of the structure of a photocell.
Using the graph, calculate the work function of the metal.
- What is the maximum speed of an emitted electron when light of wavelength 550 nm is incident on the photocell?
- Explain why a current does not flow in the photocell when the frequency of the light is less than 5.2 × 1014 Hz.
- The relationship between the current flowing in a photocell and the intensity of the light incident on the photocell was then investigated. Readings were taken and a graph was drawn to show the relationship.
Draw a sketch of the graph obtained.
- How was the intensity of the light varied?
- What conclusion about the nature of light can be drawn from these investigations?
- [2003 OL] [2002 ][2008 OL]
Give two applications of a photocell.
X-rays
- [2006][2004 OL][2007 OL][2010]What are X-rays?
- [2010 OL]Give three properties of X-rays
- [2010]How do X-rays differ from light rays?
- [2004 OL][2007 OL][2010 OL][2010]Give three uses of X-rays.
- [2006]Who discovered X-rays?
- [2002 OL][2002][2010]Draw a labelled diagram of an X-ray tube.
- [2009]How are X-rays produced?
- [2006][2005][2004 OL][2007 OL]How are the electrons emitted from the cathode?
- [2006]How are the electrons accelerated?
- [2004 OL][2007 OL]What is the function of the high voltage across the X-ray tube?
- [2004 OL][2007 OL]Name a suitable material for the target in the X-ray tube.
- [2007 OL]What happens when the electrons hit the target?
- [2002 OL]Why is a lead-shield normally put around an X-ray tube?
- [2010]
- When electrons hit the target in an X-ray tube, only a small percentage of their energy is converted into X-rays. What happens to the rest of their energy?
- How does this influence the type of target used?
- [2007 OL]Give one safety precaution when using X-rays.
- [2006]
- Calculate the kinetic energy gained by an electron when it is accelerated through a potential difference of 50 kV in an X-ray tube.
- Calculate the minimum wavelength of an X-ray emitted from the anode.
- [2010]
- A potential difference (voltage) of 40 kV is applied across an X-ray tube.
Calculate the maximum energy of an electron as it hits the target.
- Calculate the frequency of the most energetic X-ray produced.
- [2002]Justify the statement “X-ray production may be considered as the inverse of the photoelectric effect.”
- [2002 OL]
The diagram shows a simple X-ray tube.
Name the parts labelled A, B and C.- [2010 OL]What process occurs at the filament A?
- [2010 OL] Name a substance commonly used as the target B
- [2010 OL]State the function of the part marked C
Exam Solutions
- Negative charge, negligible mass , orbits nucleus, no internal structure, deflected by electric / magnetic field etc.
- Proton, neutron.
- George Stoney
- Thermionic emission is the emission of electrons from the surface of a hot metal.
- By means of an electric field and a magnetic field.
- Cathode rays are streams of high speed electrons.
- A =heating coil, B = cathode, C = anode
- The filament gets hot and thermionic emission occurs.
- A = filament/heater, B = cathode, C = anode, D = screen
- A heats the cathode.
B is the source of the electrons.
C attracts electrons.
D (fluorescent screen) detects electrons.- To attract and accelerate the beam of electrons.
- Old-style televisions/ computer monitor, X-ray machine/oscilloscope /heart monitor/ECG.
- By a the high positive voltage at the anode.
- Light is emitted (the screen fluoresces).
- It gets converted to light.
- The final kinetic energy gained by the electron equals the initial (electrical) potential energy
= 4 KeV = (4000)(1.6 × 10–19) = 6.4×10−16 J
- E = ½ mv2 Þ 6.4 ×10-16 = ½ (9.1 × 10-31)(v2) Þ v =3.75 × 107 m s-1
- F = Bev = (5 × 10–2)(1.6 × 10–19)( 3.75 × 107) = 3.0 ×10−13 N
- F = mv2/r Þ 3.0 ×10−13 = (9.1 × 10–31)( 3.75 × 107)2/r
Þ r = 4.3×10−3 m - It is the emission of electrons from the surface of a hot metal.
- Electrons
- Photoelectric Effect: Emission of electrons when light of suitable frequency falls on a metal.
Thermionic Emission: Emission of electrons from the surface of a hot metal.
- The Photoelectric Effect is the emission of electrons from a metal due to light of a suitable frequency falling upon it.
- Sound track in film, photography, counters, photocell, burglar alarm, automatic doors, etc.
- A photon is a packet of electromagnetic radiation.
- Light has a particle nature (as well as a wave nature)
- Apparatus: gold leaf electroscope with zinc plate on top, ultraviolet light source
Procedure: Charge the electroscope negatively.
Shine ultraviolet light on the zinc plate.
Observation: The leaves fall together- Electromagnetic radiation consists of packets of energy which are called quanta or photons.
- The amount of energy in each quantum could be calculated using the formula E = hf.
- The incoming radiation needs to have sufficient energy to release an electron from the surface of a metal or it won’t be absorbed at all. This amount of energy is known as the work function.
- If the incoming energy has more energy than an electron needs to be released (called its work function) then the excess energy goes to the kinetic energy of the electron.
- hf = φ + ½mv2
- E = hf
E = (6.6 × 10–34)( 3.3 × 1014) = 2.18 × 10–19 J.
- f = (4.3) eV = (4.3)( 1.6 × 10–19) J
E = hf0 Þ f0 = E/h {where E is in joules} Þ f0 = (4.3)( 1.6 × 10–19)/ 6.6 × 10–34 = 1.04 × 1015 Hz
- c = fλ Þ f = c/λ = (3.0 × 108)/(240 × 109) = 1.25 × 1015 Hz
Ek = hf - f
Ek = [(6.6 × 10–34)( 1.25 × 1015) - 1.04 × 1015)] = 1.39 × 10-19 J- Photoelectric emission occurs (electrons get emitted from the surface of the metal).
The leaves become uncharged and therefore collapse.
- Ordinary glass does not transmit UV light
- The energy associated with photons of green light is too low for the photoelectric effect does not occur, so no electrons are emitted from the electroscope.
- Any electrons emitted are attracted back to the positive electroscope.
- Some of the excess electrons flow down to the bottom and the gold leaf moves away from the main section because similar charges repel.
- Many electrons are emitted from the zinc as a result of the ultraviolet radiation shining on it. Electrons from the main part of the electroscope flow up to replace this so there aren’t as many electrons on the gold leaf to feel the repulsion.
- Infrared radiation will not have sufficient energy to release electrons so the gold leaf will not diverge to begin with.
- A is the cathode
B is the anode
- Electrons are released
- The current increases.
- A = cathode, B = anode
- Move it towards or away from the photocell.
- The brighter the light the greater the current.
- See diagram; A = cathode, B = anode
Also label glass case and vacuum inside
- The graph indicates that current only flows when the frequency of the radiation reached 5.2 × 1014 Hz, so this corresponds to the threshold frequency (f0).
f = hf0 = (6.6 × 10-34)(5.2 × 1014) = 3.432 × 10-19 J
- h(c/λ) = f + ½mv2
(6.6 × 10-34)(3 × 108/550 × 10-9) = 3.432 × 10-19 + ½ (9.1× 10-31)(v)2
v = 1.922 × 105 m s-1Because the frequency is less than the threshold frequency so does not contain enough energy to cause an electron to be released from an atom.
- See diagram. Current is directly proportional to Intensity, so a straight line graph with the line going through the origin is required.
- Vary the distance from the light source to the photocell.
- Light is made up of discrete amounts of energy called photons.
- Burglar alarm, smoke alarms, safety switch, light meters, automatic lights, counters, automatic doors, control of central heating burners, sound track in films, scanner reading bar codes, stopping conveyer belt, solar cells etc.
Specific medical use e.g. X-ray photo, photo organs, destroy cancerous cells
Specific industrial use e.g. detect cracks in metals, determine thickness of materials- High frequency electromagnetic radiation.
- Electromagnetic waves / have short wavelength, ionise, penetrate, no mass, no charge, effect photographic film, cause fluorescence, diffraction, etc.
- X-rays penetrate matter / cause ionization; light rays don’t.
- k
To photograph bones/ internal organs, to treat cancer, to detect flaws in materials.
- X-rays were discovered by Wilhelm Röntgen in 1895 (shouldn’t have got asked – not on the syllabus).
- See diagram; must include vacuum, cathode, target / anode and high (accelerating anode) voltage or H.T. / shielding / cooling / low (cathode) voltage or L.T.
- Accelerated (fast moving) electrons strike a (heavy) metal target causing electrons in the target to rise to a high orbital level. When these electrons fall back down to a lower lever they emit they energy as X-rays.
- The electrons are emitted from the cathode due to thermionic emission because the filament is hot.
- By the high voltage between the anode and cathode.
- To accelerate the electrons.
- Tungsten
- Some inner electrons in the tungsten target get bumped up to a high orbital, then quickly fall back down to a lower lever, emitting X-rays in the process.
- For protection (to prevent X-rays entering the body).
- The energy gets converted to heat.
- The target material must have (very) high melting point.
- Use a lead shield, lead apron, lead glass, monitor dosage.
- Ek (= W) = q V = (1.6 × 10-19)(50 × 103) = 8.0 × 10-15 J
- E = h c/λ
λ = [(6.6 × 10-34 )(3.0 × 108)] /(8.0 × 10-15)
- W = qV
W = (1.6 × 10-19)( 40 × 103)
W = 6.4 × 10-15 J- E = hf
f = E/h
f = (6.4 × 10-15)/(6.6 × 10-34)
f = 9.7 × 1018 Hz- X-ray: Electrons in, electromagnetic radiation is emitted.
Photoelectric: electromagnetic radiation in and electrons are emitted.
- A = Cathode / heating coil, B = Anode (target), C = Lead shield
- Thermionic emission / release of electrons // heating
- Tungsten / molybdenum
- Protection / shielding
Source : http://www.thephysicsteacher.ie/LC%20Physics/Student%20Notes/29.%20The%20Electron.doc
Web site link: http://www.thephysicsteacher.ie
Author : not indicated on the source document of the above text
If you are the author of the text above and you not agree to share your knowledge for teaching, research, scholarship (for fair use as indicated in the United States copyrigh low) please send us an e-mail and we will remove your text quickly.
Electron
Electron
Electron
This is the right place where find the answers to your questions like :
Who ? What ? When ? Where ? Why ? Which ? How ? What does Electron mean ? Which is the meaning of Electron?
Electron physics notes
Alanpedia.com from 1998 year by year new sites and innovations
Main page - Disclaimer - Contact us